What are the Mainstream Models of Inductor Functions?
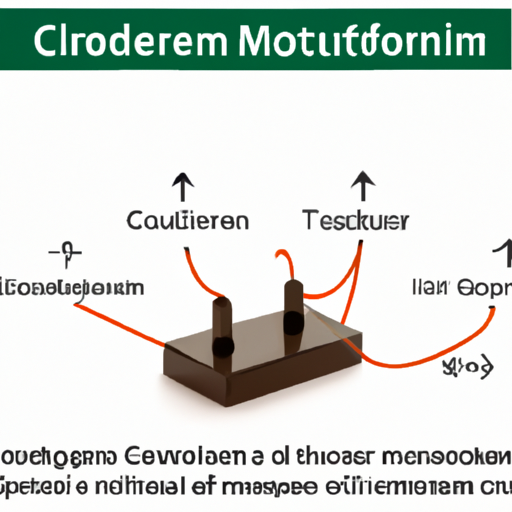
I. Introduction
Inductors are fundamental components in electrical circuits, playing a crucial role in energy storage, filtering, and signal processing. Defined as passive electrical devices that store energy in a magnetic field when electric current flows through them, inductors are essential in various applications, from power supplies to communication systems. This article aims to explore the mainstream models of inductor functions, delving into their basic principles, types, mathematical models, applications, challenges, and future trends.
II. Basic Principles of Inductance
A. Explanation of Inductance
Inductance is the property of an inductor that quantifies its ability to store energy in a magnetic field. The unit of inductance is the Henry (H), named after the American scientist Joseph Henry. When current flows through an inductor, it generates a magnetic field around it. The strength of this magnetic field is proportional to the amount of current flowing through the inductor.
B. The Role of Inductors in Energy Storage
Inductors store energy in the form of a magnetic field. When the current through the inductor changes, the magnetic field also changes, inducing a voltage that opposes the change in current, according to Lenz's Law. This property makes inductors valuable for smoothing out fluctuations in current and voltage in power supply circuits.
C. Inductor Behavior in AC and DC Circuits
In direct current (DC) circuits, inductors behave as short circuits after a brief period, allowing current to flow freely once the magnetic field is established. In alternating current (AC) circuits, however, inductors resist changes in current, leading to a phenomenon known as inductive reactance. This reactance increases with frequency, making inductors essential in filtering applications.
III. Types of Inductors
A. Air-Core Inductors
Air-core inductors are constructed without a magnetic core, relying solely on air as the medium for the magnetic field. They are often used in high-frequency applications due to their low losses and minimal distortion. However, they have lower inductance values compared to core-based inductors.
B. Iron-Core Inductors
Iron-core inductors utilize materials like ferrite or silicon steel to enhance inductance. The magnetic properties of these materials allow for greater energy storage and efficiency. They are commonly used in power transformers and inductors for power supply circuits, but they can suffer from core saturation at high currents.
C. Toroidal Inductors
Toroidal inductors are shaped like a doughnut and are known for their compact design and high efficiency. The closed-loop design minimizes electromagnetic interference and enhances inductance. They are widely used in audio equipment and power supplies.
D. Variable Inductors
Variable inductors allow for the adjustment of inductance values, making them useful in tuning circuits, such as radio frequency (RF) applications. They can be adjusted mechanically or electronically, providing flexibility in circuit design.
IV. Mathematical Models of Inductors
A. Basic Inductance Formula
The inductance (L) of an inductor can be expressed using the formula:
\[ L = \frac{NΦ}{I} \]
Where:
- \( L \) is the inductance in Henries,
- \( N \) is the number of turns in the coil,
- \( Φ \) is the magnetic flux in Webers,
- \( I \) is the current in Amperes.
This formula highlights the relationship between the physical characteristics of the inductor and its inductance.
B. Series and Parallel Inductance Calculations
Inductors can be connected in series or parallel, affecting the total inductance in a circuit. For series connections, the total inductance is the sum of individual inductances:
\[ L_{total} = L_1 + L_2 + L_3 + ... \]
For parallel connections, the total inductance can be calculated using the formula:
\[ \frac{1}{L_{total}} = \frac{1}{L_1} + \frac{1}{L_2} + \frac{1}{L_3} + ... \]
C. Impedance of Inductors in AC Circuits
In AC circuits, inductors exhibit reactance, which is a measure of their opposition to current changes. The inductive reactance (\( X_L \)) is given by:
\[ X_L = 2πfL \]
Where \( f \) is the frequency of the AC signal. This reactance leads to phase shifts between voltage and current, which is critical in RLC (resistor-inductor-capacitor) circuit analysis.
V. Inductor Models in Circuit Simulation
A. SPICE Models
SPICE (Simulation Program with Integrated Circuit Emphasis) is a widely used simulation software for electronic circuits. Inductors in SPICE are represented as ideal components, but real-world behavior can be modeled by incorporating parasitic elements.
B. Behavioral Models
Behavioral models differentiate between ideal and non-ideal inductors. Ideal inductors have no resistance or capacitance, while non-ideal inductors include parasitic elements that affect performance. These models are crucial for accurate circuit simulations.
C. Advanced Modeling Techniques
Finite Element Analysis (FEA) is an advanced modeling technique used to analyze the electromagnetic behavior of inductors, especially in high-frequency applications. FEA allows engineers to optimize inductor designs for performance and efficiency.
VI. Applications of Inductors
A. Power Supply Circuits
Inductors play a vital role in power supply circuits, where they are used for filtering and energy storage. They smooth out voltage fluctuations, ensuring stable power delivery to electronic devices.
B. RF and Communication Systems
In RF applications, inductors are used in oscillators and tuners to select specific frequencies. Their ability to store and release energy makes them essential for signal modulation and demodulation.
C. Audio Equipment
Inductors are commonly found in audio equipment, where they are used in crossovers and equalizers. They help filter out unwanted frequencies, ensuring high-quality sound reproduction.
D. Inductors in Renewable Energy Systems
In renewable energy systems, such as wind and solar, inductors are used in inverters and converters to manage energy flow and improve efficiency. Their role in energy storage is crucial for balancing supply and demand.
VII. Challenges and Limitations
A. Core Saturation
Core saturation occurs when the magnetic core of an inductor reaches its maximum magnetic flux density, leading to a decrease in inductance and potential circuit failure. This phenomenon is particularly concerning in high-current applications.
B. Parasitic Effects
Inductors are subject to parasitic resistance and capacitance, which can affect their performance. These parasitic elements can lead to energy losses and distortions in circuit behavior, necessitating careful design considerations.
C. Temperature Effects
Temperature variations can influence the inductance and resistance of inductors. As temperature increases, resistance typically rises, which can impact the efficiency and performance of the inductor in a circuit.
VIII. Future Trends in Inductor Technology
A. Miniaturization and Integration
The trend toward miniaturization in electronics has led to the development of surface-mount technology (SMT) inductors. These compact inductors are essential for modern devices, where space is at a premium.
B. New Materials and Designs
Research into new materials, such as superconductors, is paving the way for more efficient inductors. These materials can significantly reduce energy losses and improve performance in high-frequency applications.
C. Smart Inductors
The integration of inductors with IoT (Internet of Things) and smart grid technologies is an emerging trend. Smart inductors can provide real-time monitoring and control, enhancing the efficiency of electrical systems.
IX. Conclusion
Inductors are indispensable components in modern electronics, serving various functions across multiple applications. From energy storage to filtering and signal processing, their importance cannot be overstated. As technology advances, the development of new materials, designs, and integration with smart technologies will continue to shape the future of inductors. Understanding the mainstream models of inductor functions is essential for engineers and designers as they navigate the complexities of modern electronic systems.
X. References
1. Paul, C. R. (2008). *Introduction to Electromagnetic Compatibility*. Wiley.
2. Sedra, A. S., & Smith, K. C. (2015). *Microelectronic Circuits*. Oxford University Press.
3. Razavi, B. (2016). *RF Microelectronics*. Prentice Hall.
4. Horowitz, P., & Hill, W. (2015). *The Art of Electronics*. Cambridge University Press.
5. SPICE User's Manual. (2020). *University of California, Berkeley*.
This blog post provides a comprehensive overview of the mainstream models of inductor functions, covering essential principles, types, mathematical models, applications, challenges, and future trends. Each section is designed to inform and engage readers, offering insights into the critical role inductors play in modern electronics.
What are the Mainstream Models of Inductor Functions?
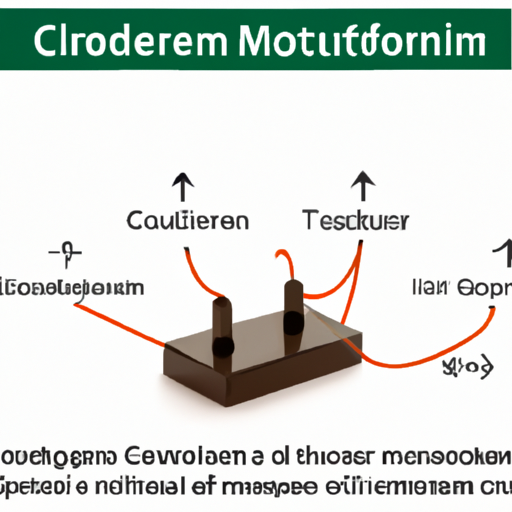
I. Introduction
Inductors are fundamental components in electrical circuits, playing a crucial role in energy storage, filtering, and signal processing. Defined as passive electrical devices that store energy in a magnetic field when electric current flows through them, inductors are essential in various applications, from power supplies to communication systems. This article aims to explore the mainstream models of inductor functions, delving into their basic principles, types, mathematical models, applications, challenges, and future trends.
II. Basic Principles of Inductance
A. Explanation of Inductance
Inductance is the property of an inductor that quantifies its ability to store energy in a magnetic field. The unit of inductance is the Henry (H), named after the American scientist Joseph Henry. When current flows through an inductor, it generates a magnetic field around it. The strength of this magnetic field is proportional to the amount of current flowing through the inductor.
B. The Role of Inductors in Energy Storage
Inductors store energy in the form of a magnetic field. When the current through the inductor changes, the magnetic field also changes, inducing a voltage that opposes the change in current, according to Lenz's Law. This property makes inductors valuable for smoothing out fluctuations in current and voltage in power supply circuits.
C. Inductor Behavior in AC and DC Circuits
In direct current (DC) circuits, inductors behave as short circuits after a brief period, allowing current to flow freely once the magnetic field is established. In alternating current (AC) circuits, however, inductors resist changes in current, leading to a phenomenon known as inductive reactance. This reactance increases with frequency, making inductors essential in filtering applications.
III. Types of Inductors
A. Air-Core Inductors
Air-core inductors are constructed without a magnetic core, relying solely on air as the medium for the magnetic field. They are often used in high-frequency applications due to their low losses and minimal distortion. However, they have lower inductance values compared to core-based inductors.
B. Iron-Core Inductors
Iron-core inductors utilize materials like ferrite or silicon steel to enhance inductance. The magnetic properties of these materials allow for greater energy storage and efficiency. They are commonly used in power transformers and inductors for power supply circuits, but they can suffer from core saturation at high currents.
C. Toroidal Inductors
Toroidal inductors are shaped like a doughnut and are known for their compact design and high efficiency. The closed-loop design minimizes electromagnetic interference and enhances inductance. They are widely used in audio equipment and power supplies.
D. Variable Inductors
Variable inductors allow for the adjustment of inductance values, making them useful in tuning circuits, such as radio frequency (RF) applications. They can be adjusted mechanically or electronically, providing flexibility in circuit design.
IV. Mathematical Models of Inductors
A. Basic Inductance Formula
The inductance (L) of an inductor can be expressed using the formula:
\[ L = \frac{NΦ}{I} \]
Where:
- \( L \) is the inductance in Henries,
- \( N \) is the number of turns in the coil,
- \( Φ \) is the magnetic flux in Webers,
- \( I \) is the current in Amperes.
This formula highlights the relationship between the physical characteristics of the inductor and its inductance.
B. Series and Parallel Inductance Calculations
Inductors can be connected in series or parallel, affecting the total inductance in a circuit. For series connections, the total inductance is the sum of individual inductances:
\[ L_{total} = L_1 + L_2 + L_3 + ... \]
For parallel connections, the total inductance can be calculated using the formula:
\[ \frac{1}{L_{total}} = \frac{1}{L_1} + \frac{1}{L_2} + \frac{1}{L_3} + ... \]
C. Impedance of Inductors in AC Circuits
In AC circuits, inductors exhibit reactance, which is a measure of their opposition to current changes. The inductive reactance (\( X_L \)) is given by:
\[ X_L = 2πfL \]
Where \( f \) is the frequency of the AC signal. This reactance leads to phase shifts between voltage and current, which is critical in RLC (resistor-inductor-capacitor) circuit analysis.
V. Inductor Models in Circuit Simulation
A. SPICE Models
SPICE (Simulation Program with Integrated Circuit Emphasis) is a widely used simulation software for electronic circuits. Inductors in SPICE are represented as ideal components, but real-world behavior can be modeled by incorporating parasitic elements.
B. Behavioral Models
Behavioral models differentiate between ideal and non-ideal inductors. Ideal inductors have no resistance or capacitance, while non-ideal inductors include parasitic elements that affect performance. These models are crucial for accurate circuit simulations.
C. Advanced Modeling Techniques
Finite Element Analysis (FEA) is an advanced modeling technique used to analyze the electromagnetic behavior of inductors, especially in high-frequency applications. FEA allows engineers to optimize inductor designs for performance and efficiency.
VI. Applications of Inductors
A. Power Supply Circuits
Inductors play a vital role in power supply circuits, where they are used for filtering and energy storage. They smooth out voltage fluctuations, ensuring stable power delivery to electronic devices.
B. RF and Communication Systems
In RF applications, inductors are used in oscillators and tuners to select specific frequencies. Their ability to store and release energy makes them essential for signal modulation and demodulation.
C. Audio Equipment
Inductors are commonly found in audio equipment, where they are used in crossovers and equalizers. They help filter out unwanted frequencies, ensuring high-quality sound reproduction.
D. Inductors in Renewable Energy Systems
In renewable energy systems, such as wind and solar, inductors are used in inverters and converters to manage energy flow and improve efficiency. Their role in energy storage is crucial for balancing supply and demand.
VII. Challenges and Limitations
A. Core Saturation
Core saturation occurs when the magnetic core of an inductor reaches its maximum magnetic flux density, leading to a decrease in inductance and potential circuit failure. This phenomenon is particularly concerning in high-current applications.
B. Parasitic Effects
Inductors are subject to parasitic resistance and capacitance, which can affect their performance. These parasitic elements can lead to energy losses and distortions in circuit behavior, necessitating careful design considerations.
C. Temperature Effects
Temperature variations can influence the inductance and resistance of inductors. As temperature increases, resistance typically rises, which can impact the efficiency and performance of the inductor in a circuit.
VIII. Future Trends in Inductor Technology
A. Miniaturization and Integration
The trend toward miniaturization in electronics has led to the development of surface-mount technology (SMT) inductors. These compact inductors are essential for modern devices, where space is at a premium.
B. New Materials and Designs
Research into new materials, such as superconductors, is paving the way for more efficient inductors. These materials can significantly reduce energy losses and improve performance in high-frequency applications.
C. Smart Inductors
The integration of inductors with IoT (Internet of Things) and smart grid technologies is an emerging trend. Smart inductors can provide real-time monitoring and control, enhancing the efficiency of electrical systems.
IX. Conclusion
Inductors are indispensable components in modern electronics, serving various functions across multiple applications. From energy storage to filtering and signal processing, their importance cannot be overstated. As technology advances, the development of new materials, designs, and integration with smart technologies will continue to shape the future of inductors. Understanding the mainstream models of inductor functions is essential for engineers and designers as they navigate the complexities of modern electronic systems.
X. References
1. Paul, C. R. (2008). *Introduction to Electromagnetic Compatibility*. Wiley.
2. Sedra, A. S., & Smith, K. C. (2015). *Microelectronic Circuits*. Oxford University Press.
3. Razavi, B. (2016). *RF Microelectronics*. Prentice Hall.
4. Horowitz, P., & Hill, W. (2015). *The Art of Electronics*. Cambridge University Press.
5. SPICE User's Manual. (2020). *University of California, Berkeley*.
This blog post provides a comprehensive overview of the mainstream models of inductor functions, covering essential principles, types, mathematical models, applications, challenges, and future trends. Each section is designed to inform and engage readers, offering insights into the critical role inductors play in modern electronics.